Evolution of the Universe – The Big Bang
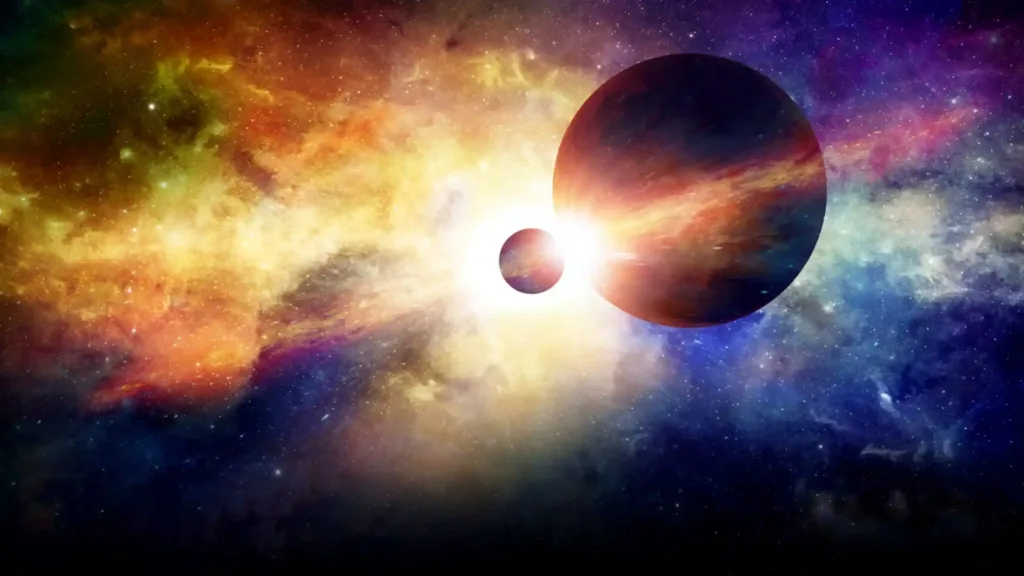
The Big Bang Theory is the most widely accepted explanation for the origin and evolution of the universe. It describes the universe’s beginning from an extremely hot, dense state, followed by its continuous expansion. Over the decades, Big Bang model has been enriched and expanded with evidence from various scientific disciplines such as cosmology, astronomy, and particle physics. This detailed exploration of the Big Bang will cover its mechanisms, consequences, and the critical roles of concepts like dark matter, dark energy, cosmic microwave background (CMB), galaxy formation, and more. We will also delve into unresolved mysteries in cosmology, such as the flatness problem, horizon problem, and baryogenesis.
What is the Big Bang?
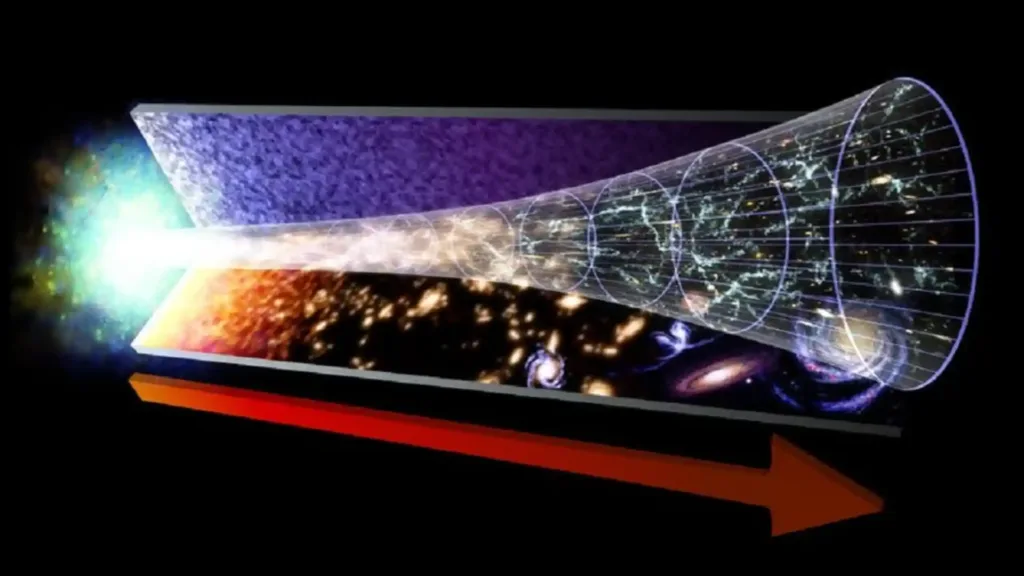
The Big Bang theory posits that about 13.8 billion years ago, the universe began from a singularity — a point of infinite density and temperature. This singularity rapidly expanded in an event known as the Big Bang, setting the universe into motion. Over time, the universe cooled down, leading to the formation of subatomic particles and simple elements, eventually giving rise to galaxies, stars, and planets.
The Big Bang is not a traditional explosion; rather, it represents the rapid expansion of space itself. Before the Big Bang, the concept of “before” becomes meaningless since time and space as we understand them were nonexistent.
The term “Big Bang” was coined by British scientist Fred Hoyle during a 1949 radio broadcast. Despite its name, the Big Bang was not an explosion in the conventional sense but rather an expansion of space itself.
Key observations supporting the Big Bang Theory :
Cosmic Microwave Background (CMB)
One of the most critical pieces of evidence supporting the Big Bang theory is the cosmic microwave background (CMB). Discovered in 1965, the CMB is the faint afterglow of the Big Bang, a snapshot of the universe when it was just 380,000 years old. At this stage, the universe had cooled down enough for protons and electrons to combine and form neutral hydrogen atoms. This “decoupling” of matter and radiation allowed light to travel freely, and today, we observe this radiation in the microwave spectrum.
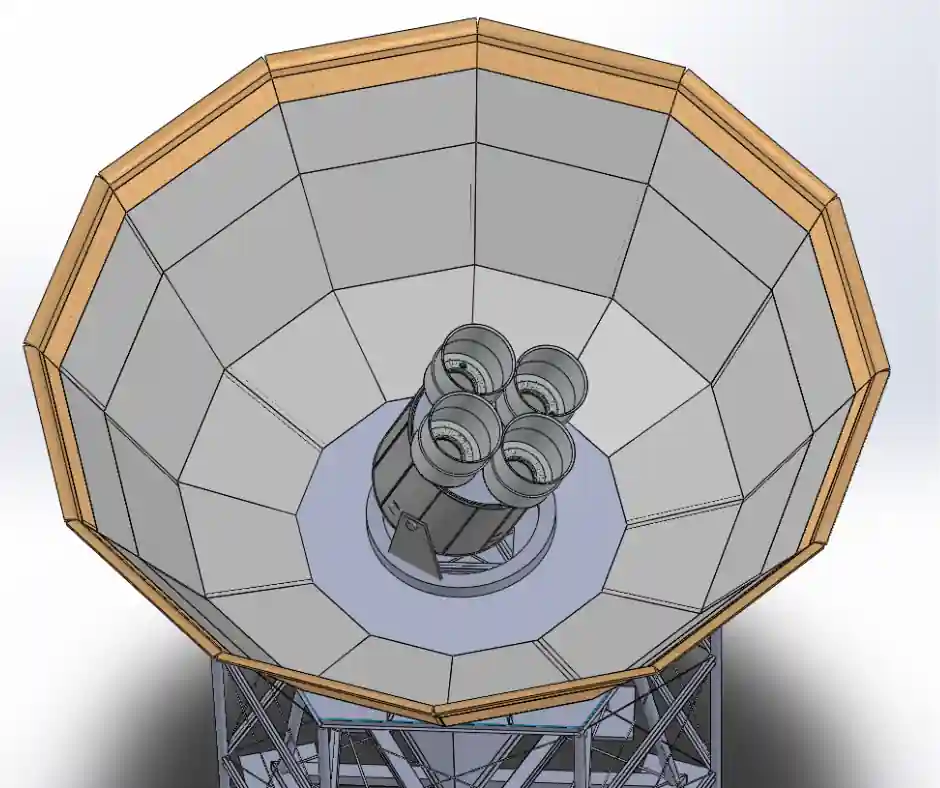
The BICEP2 telescope and other advanced observatories have provided increasingly detailed measurements of the CMB. By analyzing absorption lines and the spectra of Quasars, scientists can infer conditions in the early universe and verify Big Bang predictions.
Galaxy Formation and Evolution
Understanding the process of Galaxy formation is key to understanding the broader structure of the universe. The Hubble expansion shows that galaxies are moving away from each other, implying that they were once closer together. The early universe was composed of primordial gas clouds — primarily hydrogen and helium, formed during Big Bang nucleosynthesis (BBN).
Within these clouds, the first stars (Population II stars) ignited through star formation, forming the first galaxies. These galaxies later merged into larger structures such as galaxy clusters and superclusters. The study of distant galaxies helps us understand how galaxies evolve over time.
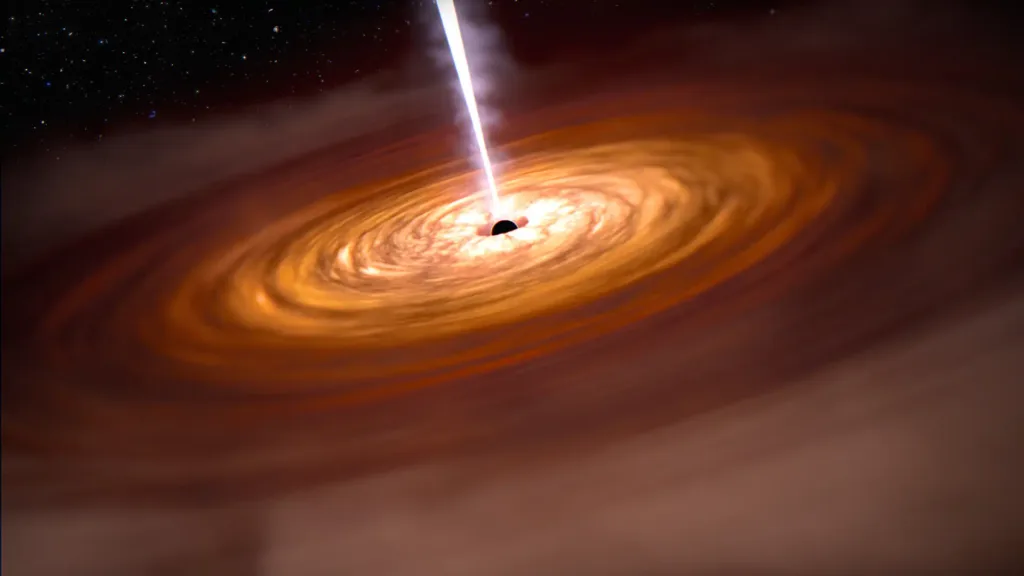
Quasars, which are extremely bright objects powered by supermassive black holes, serve as “lighthouses” of the distant universe. They provide crucial insights into the structure formation of the early cosmos. Observations of quasar spectra have also revealed the presence of heavy elements, which were formed in the cores of the first stars.
The Lambda-CDM Model and Dark Matter
The most successful cosmological model to date is the Lambda-CDM (ΛCDM) model, which describes the universe as being composed of cold dark matter (CDM) and dark energy (Lambda). This model is consistent with observations of the CMB, gravitational lensing, and baryon acoustic oscillations.
Dark matter is a mysterious substance that does not emit or absorb light but exerts gravitational forces. While it constitutes about 85% of the total matter in the universe, it remains undetected by traditional means. Cold dark matter model explains the formation of galaxies and large-scale structures, but unresolved problems like the dwarf galaxy problem and the cuspy halo problem remain.
Characteristics of Dark Matter:
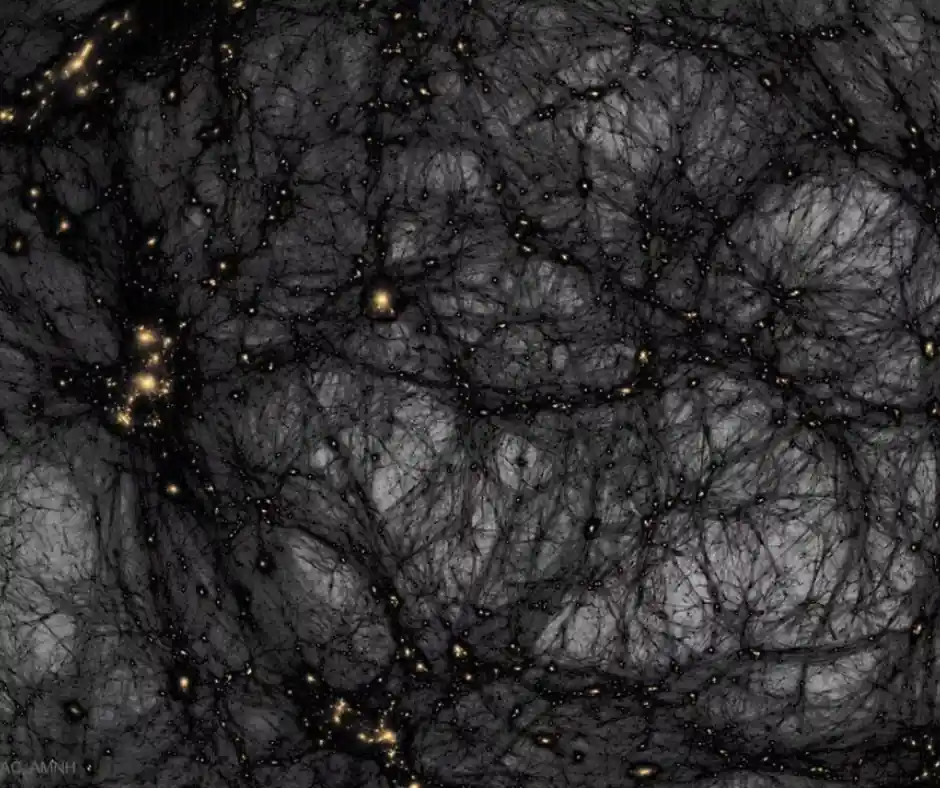
- Gravitational Influence: Dark matter exerts gravitational forces on galaxies and galaxy clusters, affecting their rotation curves and the motion of galaxies within clusters.
- Non-Baryonic Nature: Unlike ordinary matter, dark matter is not composed of protons and neutrons. It is hypothesized to consist of exotic particles, such as Weakly Interacting Massive Particles (WIMPs) or axions.
- Detection Efforts: Efforts to detect dark matter directly include underground laboratories searching for WIMPs and indirect detection through observing high-energy particles or gamma rays that might result from dark matter interactions.
Dark Energy and the Accelerating Universe
In 1998, observations of Type Ia supernovae led to the discovery that the universe’s expansion is accelerating. This acceleration is attributed to a force known as dark energy, which makes up about 70% of the universe’s total energy content. Einstein’s cosmological constant is the simplest explanation for dark energy, though other models, such as quintessence, have also been proposed.
The redshift–magnitude relation of distant supernovae provided the first clear evidence of the universe’s accelerated expansion. The nature of dark energy remains one of the biggest mysteries in cosmology, as it challenges our understanding of the fundamental forces of nature.
Characteristics of Dark Energy:
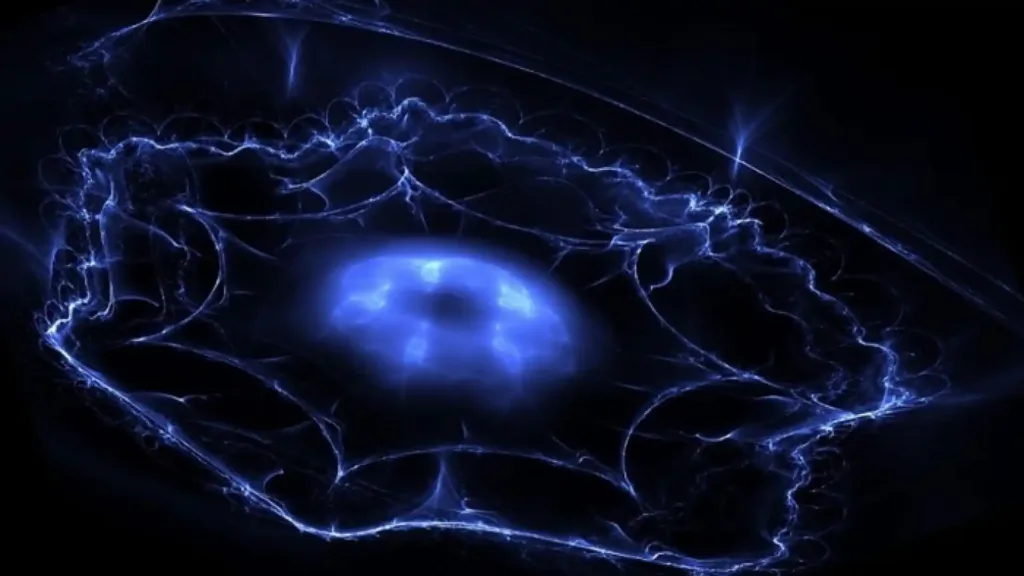
- Cosmological Constant: One theory suggests that dark energy is related to the cosmological constant, a term introduced by Albert Einstein in his field equations of General Relativity, representing a constant energy density filling space homogeneously.
- Equation of State: The equation of state of dark energy, which describes how its pressure and density relate, has been estimated to be approximately -1, consistent with the cosmological constant hypothesis.
- Observational Evidence: Evidence for dark energy comes from several sources, including observations of supernovae, the large-scale structure of the universe, and the CMB. These observations indicate that dark energy constitutes about 68% of the universe.
Primordial Gravitational Waves and Inflation
The inflation theory was introduced to solve key problems in the Big Bang model, such as the horizon problem, flatness problem, and magnetic monopole problem. According to inflation, the universe underwent an extremely rapid expansion during the first 10^-36 to 10^-32 seconds after the Big Bang. This rapid expansion smoothed out the universe’s curvature and explains why the cosmological parameter Omega (Ω) is so close to 1, solving the flatness problem.
Primordial gravitational waves are ripples in spacetime generated by inflation. Detecting these waves would provide direct evidence for inflation. Gravitational-wave observatories, such as LIGO and Virgo, are currently searching for signals from these waves.
Characteristics of Primordial Gravitational Waves:
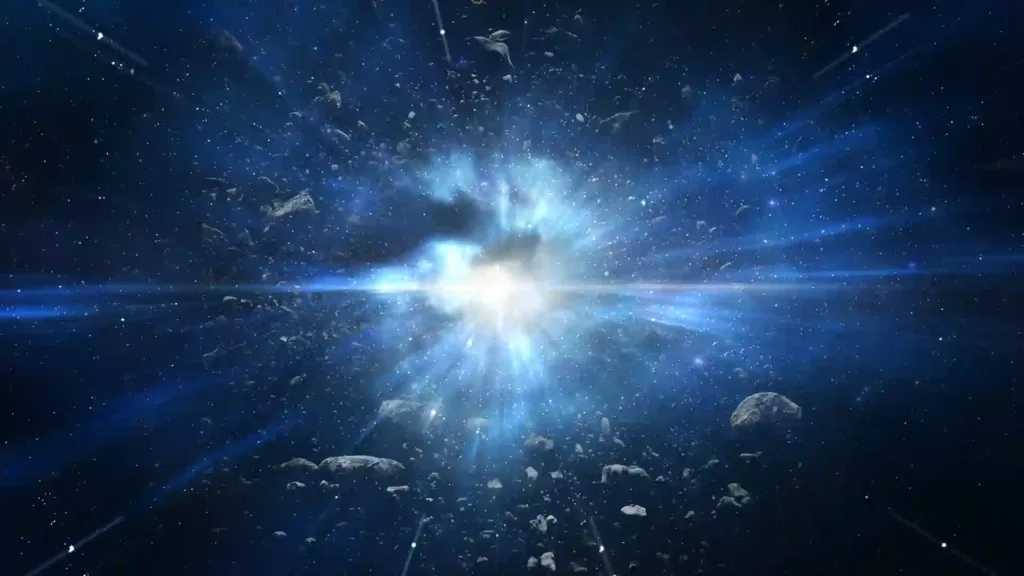
- Inflationary Theory: During inflation, quantum fluctuations were stretched to macroscopic scales, creating gravitational waves that left an imprint on the CMB.
- Detection and Significance: Detecting these waves directly would provide critical evidence for inflationary theory and offer insights into the very early universe’s conditions. Efforts to detect primordial gravitational waves include observing the B-mode polarization of the CMB.
Baryogenesis and the Matter-Antimatter Asymmetry
The universe today is composed predominantly of matter, despite the fact that the Big Bang should have produced equal amounts of matter and antimatter. This discrepancy is known as the Baryon asymmetry problem. According to Sakharov conditions, a set of criteria must be met for this asymmetry to arise. The process by which more matter than antimatter was produced is called baryogenesis.
Key Aspects of Matter-Antimatter Asymmetry:
- Annihilation: When matter and anti-matter meet, they annihilate each other, releasing energy. If matter and anti-matter were created in equal amounts, they would annihilate completely, leaving a universe devoid of matter. However, we observe a matter-dominated universe.
- CP Violation: CP (Charge Parity) violation refers to the lack of symmetry between particles and antiparticles. Observations of CP violation in certain particle decays suggest that the standard model might not fully account for the observed asymmetry, leading to new physics beyond the standard model.
- Experimental Evidence: Experiments studying the decays of particles such as the kaon and B mesons have provided evidence of CP violation, but it is still insufficient to fully explain the observed matter-antimatter imbalance.
Key Aspects of Baryogenesis:
- Sakharov Conditions: Andrei Sakharov proposed three necessary conditions for baryogenesis: a violation of baryon number conservation, C and CP symmetry violations, and interactions out of equilibrium. These conditions provide a framework for understanding how a slight excess of matter over anti-matter could have occurred.
- Theoretical Models: Various models, including those involving the electroweak phase transition or leptogenesis, attempt to explain the origin of this asymmetry. Leptogenesis, for instance, posits that an excess of leptons over anti-leptons could eventually lead to a matter-antimatter asymmetry.
Many theories, including those based on Grand Unified Theories (GUTs) and electroweak symmetry breaking, attempt to explain baryogenesis, but the exact mechanism remains unknown.
The Future of the Universe
Several theories predict the ultimate fate of the universe. The most likely scenarios include the heat death, where the universe continues expanding and eventually reaches thermodynamic equilibrium, or the Big Crunch, where the universe’s expansion reverses, causing it to collapse back into a singularity.
Another possibility is the Big Rip, where dark energy tears apart galaxies, stars, and even atoms. The Planck time, the smallest measurable unit of time, marks the limit of our understanding of the universe’s future and past.
Big Rip : End of the Universe
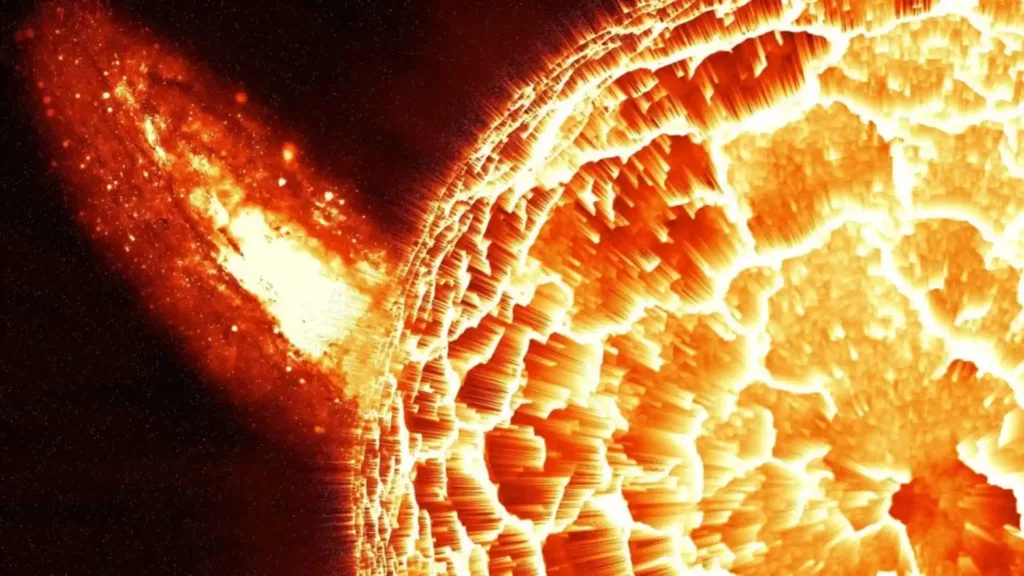
The Big Rip is a hypothetical scenario for the ultimate fate of the universe, proposed as an alternative to the Big Freeze or Big Crunch. It arises from the idea that the accelerated expansion of the universe, driven by dark energy, could eventually become so extreme that it leads to a dramatic disintegration of cosmic structures.
Concept of the Big Rip
1. Dark Energy and Accelerated Expansion:
The Big Rip is based on the influence of dark energy, a mysterious form of energy responsible for the accelerated expansion of the universe. Observations, such as those of distant supernovae and the cosmic microwave background radiation, suggest that the expansion rate of the universe is increasing. Dark energy is thought to be driving this acceleration.
2. Mathematical Framework:
The Big Rip scenario is modeled using equations from General Relativity and cosmological models, particularly those involving dark energy. It requires a specific type of dark energy with an equation of state where the parameter www (representing the ratio of pressure to energy density) is less than -1. This kind of dark energy is often referred to as “phantom energy.”
3. Phases Leading to the Big Rip: The Big Rip involves several stages as the universe expands:
- Galactic Scale: In the early stages, the expansion rate accelerates, causing galaxies to move further apart. Eventually, the gravitational bonds holding galaxies together could be overcome by the expansion force.
- Solar System Scale: As the expansion continues to accelerate, the force can become strong enough to tear apart solar systems. Stars and planets within galaxies could be pulled apart as the fabric of space stretches.
- Planetary Scale: On even smaller scales, the expansion force becomes strong enough to disrupt the bonds between atoms, leading to the disintegration of planets and other celestial objects.
- Atomic and Subatomic Scales: In the final stages, the expansion would overcome the fundamental forces holding atoms and subatomic particles together, ultimately ripping apart all matter.
4. Timeline and Predictions:
The timeline for the Big Rip depends on the nature of dark energy and its properties. If dark energy has the properties predicted by the phantom energy model, the Big Rip could occur within a few billion years from now. The exact timing is uncertain and depends on future observational data.
5. Observational Evidence:
Currently, there is no direct observational evidence for the Big Rip. Instead, it is a theoretical outcome based on our understanding of dark energy and its potential effects on cosmic expansion. Future observations and measurements of the universe’s expansion rate, dark energy properties, and cosmic structure will help refine our understanding and either support or refute the Big Rip scenario.
Comparison with Other Scenarios
1. Big Freeze (Heat Death):
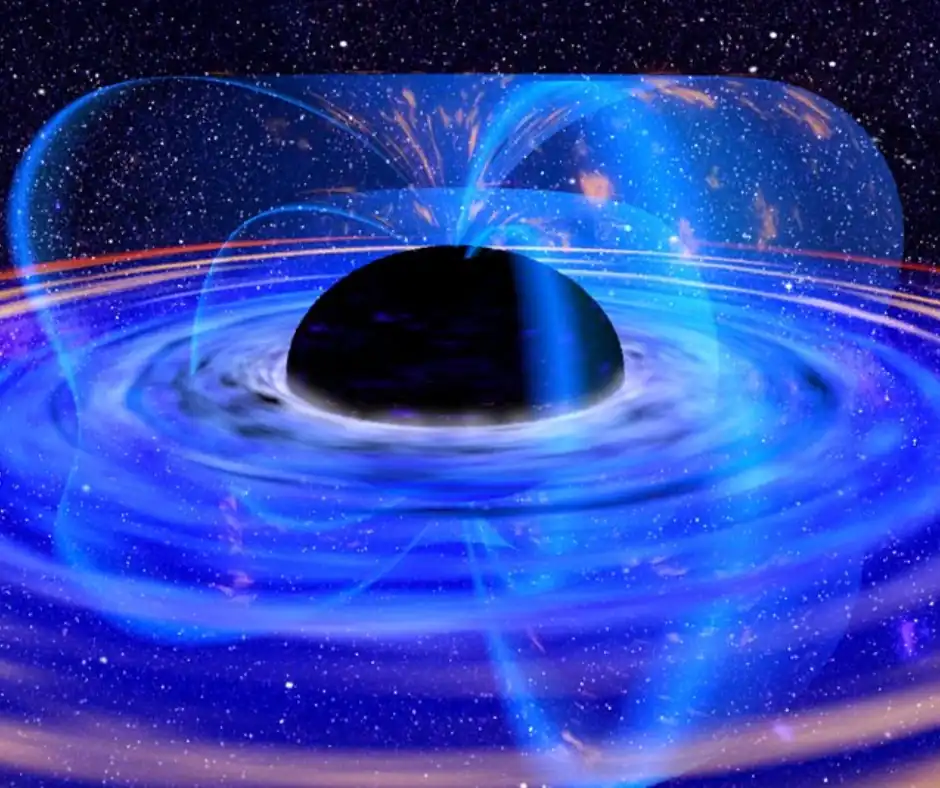
In the Big Freeze scenario, the universe continues to expand at an accelerated rate, but the rate of expansion is not sufficient to tear apart galaxies, stars, or matter itself. Over time, the universe becomes increasingly cold and dark as stars burn out and galaxies drift apart, leading to a state of maximum entropy.
2. Big Crunch:
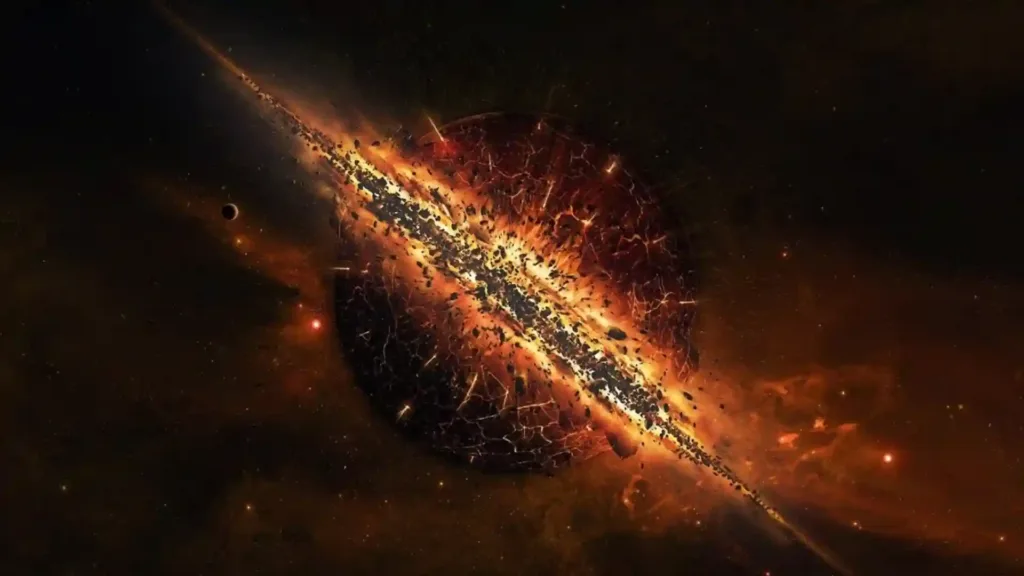
The Big Crunch is a scenario where the expansion of the universe eventually slows down and reverses, leading to a contraction. The universe would collapse back into a hot, dense state, potentially ending in a singularity. This scenario requires a deceleration of expansion and is less likely given current observations suggesting accelerated expansion.
The Big Bang Theory has revolutionized our understanding of the universe. From the initial singularity and rapid inflation to the formation of galaxies and the mysterious forces of dark matter and dark energy, the story of the universe is far from complete. As gravitational-wave observatories, primordial gas clouds, and increasingly detailed observations of quasars and the CMB continue to provide new data, the mysteries of the universe’s origin and fate may one day be fully unraveled. Until then, the Big Bang remains the most compelling narrative of how everything began, providing a cosmic roadmap that spans from the Planck time to the heat death.
Details in the Articles are collected from trusted Sources you can visit Wikipedia for more details.